Quark-Gluon Plasma Simulation Reveals Surprising Complexity
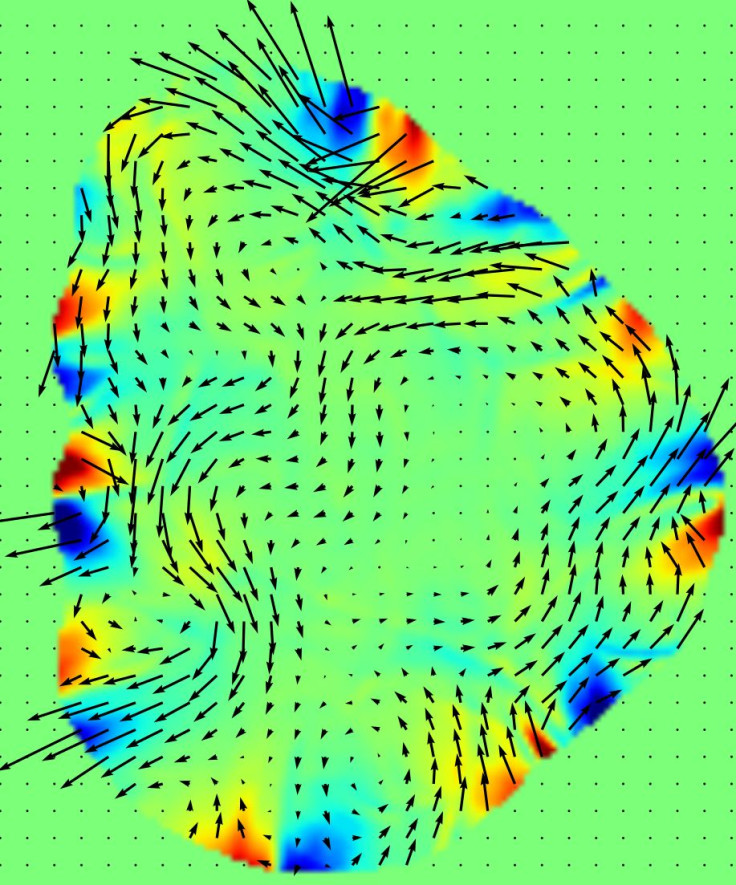
When our universe burst into existence approximately 13.8 billion years ago, it initially gave birth to a “primordial soup” composed of a state of matter known as the quark-gluon plasma. This plasma, which vanished after a few millionths of a second, is believed to have existed at extremely high temperatures and densities — conditions in which regular matter cannot exist.
Physicists believe understanding the nature of quark-gluon plasma is key to not only comprehending the earliest moments of our universe — which no telescope can ever reveal — but also in formulating equations that explain the behavior of the strong nuclear force, which holds the quarks in a nucleus together.
However, while scientists have been successful in recreating this exotic soup during high-energy collisions in particle accelerators like the Relativistic Heavy Ion Collider (RHIC) and the Large Hadron Collider (LHC), we still do not have a comprehensive understanding of the inner workings of this ephemeral plasma state.
An international team of researchers has now created powerful supercomputer simulations of colliding atomic nuclei in order to obtain fresh insights into the structure of quark-gluon plasma. Their experiments, detailed in a study published in the latest issue of the journal Physical Review Letters, reveal that the inner structure of the plasma is surprisingly complex.
“In our sophisticated simulations, we found that there is much more structure to this plasma than we realized,” study co-author Xin-Nian Wang from the Lawrence Berkeley National Laboratory in California, said in a statement.
The simulations produced structures shaped like rings and vortices, which the researchers believe were created due to the angular momentum of the colliding nuclei being transferred to the quark-gluon plasma. In addition, the simulations also revealed outward flow from hotspots in the plasma that resemble the spokes of a wheel — a phenomenon that causes the wobbling superfluid to expand from its middle more rapidly than from the top.
These findings, the researchers said, were “completely unexpected.”
“We can think about this as opening a completely new window of looking at quark-gluon plasmas, and how to study them,” Wang said. “Hopefully this will provide another gateway into understanding why this quark-gluon fluid is such a perfect fluid — the nature of why this is so is still a puzzle. This work will benefit not only theory, but also experiments.”
Experiments would now have to be carried out at particle accelerators such as the RHIC and the LHC to check if the simulation accurately predicts the behavior of quark-gluon plasma.
“Wang and his collaborators have developed a sophisticated, state-of-the-art hydrodynamic model of the quark-gluon plasma and have identified swirling structures that vary within the fluid itself,” Michael Lisa, a physics professor at Ohio State University, who was not involved in the study, said in the statement. “Even more useful is the fact that they propose a method to measure these structures in the laboratory.”
© Copyright IBTimes 2024. All rights reserved.